David B. Durham, MD
The year is 1848. A very small blurb in the Free Soil Union, a small Vermont newspaper, published what would be the case of traumatic brain injury most often taught in medical schools for the next one hundred years. It reads as follows:
"Horrible Accident, Phineas P. Gage, a foreman on the Rutland Railroad at Cavendish, Vermont, was tamping for a blast on Wednesday last, when the powder exploded, carrying through his head an iron instrument, an inch and a fourth in circumference, and three feet and eight inches in length. The iron entered on the side of his face, shattering the upper jaw, and passing back of the left eye, and out the top of his head. Singularly enough, he was alive at two o'clock the next afternoon, in full possession of his reason, and free from pain.
Gage was attended to immediately by the town’s physician, Dr. John Martin Harlow, who, to his amazement, found Gage to be “in full possession of his reason.” Now let us pause for a moment to consider what has been proven to be historical fact: 1. An “iron instrument, an inch and a fourth in circumference, and three feet and eight inches in length” passed quickly and completely through the subject’s head; 2. He survived; 3. He was able to function sufficiently well enough to travel to, and work in, New York, Chile and San Francisco (proven by confirmed review of historical documents). Below is the only known photograph of Gage in existence, holding the railroad spike that famously traversed his brain. It is followed by a photograph of his skull after his death (in 1868) and exhumation of his remains, as well as a drawing and computerized image of the rail tie as it passed through his brain. The question left unanswered regarding life after his injury—was Phineas Gage still, well, Phineas Gage? A report by Dr. Harlow, twenty years after the event, documented the “mental manifestations” of Gage’s brain injury in the Bulletin of the Massachusetts Medical Society, and gave a public account of what his family and friends were already well aware.
Phineas P. Gage
1823–1868
S
Dramatic Changes
To his wife, family and those close to him, Phineas P. Gage was clearly far from the same person they once knew. Historical records indicate they began to notice dramatic changes in his personality almost immediately. An excerpt from Dr. Harlow’s 1868 report essentially summarizes what everyone who knew Gage had concluded many years before:
His contractors, who regarded him as the most efficient and capable foreman in their employ previous to his injury, considered the change in his mind so marked that they could not give him his place again. He is fitful, irreverent, indulging at times in the grossest profanity (which was not previously his custom), manifesting but little deference for his fellows, impatient of restraint of advice when it conflicts with his desires, at times pertinaciously obstinent, yet capricious and vacillating, devising many plans of future operation, which are no sooner arranged than they are abandoned in turn for others appearing more feasible. In this regard, his mind was radically changed, so decidedly that his friends and acquaintances said he was "no longer Gage."
Phineas Gage gave us the first well-documented account of a frontal lobotomy. The rail tie dissected the fibers connecting both hemispheres of the frontal lobe of his brain, an area responsible for both personality and “executive control,” effectively severing communication between the left and right frontal hemispheres. Executive control is the brain’s ability to plan, sequence and initiate purposeful present and future actions with the proper degree of impulse control (aggression vs. non-aggression, exercising patience, flight vs. fight, etc.). Personality, albeit an amalgamation of the functions of many areas of the brain, has its epicenter in the orbitofrontal cortex (OFC) —located essentially right between your eyebrows, at the “tip” of the brain’s frontal lobe. Expression of language is a function of a small (dime-size) area in the left frontal lobe (in the majority of people). Thus, the frontal lobe is what allows us to operate in a manner that is socially acceptable. If you have ever been around a child with ADHD, and witnessed the hyperactivity and impaired attention span, you have witnessed a frontal lobe disorder first hand.
Brain function, and injury, can be understood using a few basic analogies: 1. The brain can be compared to a giant computer circuit board with millions upon millions of micro-connections folded in layers like a lasagna; 2. Those connections are akin to spider webs—resilient but breakable; 3. New webs can be spun, and old webs can often be repaired, but the more of them that are severed, the further from your old self you will be. Impairment of executive control from frontal lobe damage is one of the most direct manifestations of brain injury. The frontal lobe is the largest lobe in the brain, but there are five lobes in total (the fifth is the uncus, which is often not reported as a lobe). The frontal lobe is given an array of responsibilities, most of which are related to fine-tuning our actions that may originate in the other regions of the brain. This “fine tuning” gives us the ability to manage more subtle social interactions, including intimacy and aggression. Each of the five anatomical regions of the brain is responsible for a few of our most basic functions. Following are a few examples of what can result from damage to each of the four major regions.
Why Problems Might Occur
The temporal lobes of the brain (right and left) are generally understood as being responsible for the meaningful relay of memory as well as for learning new things. The left temporal lobe also contains a small (dime-sized) region responsible in most people for the understanding of language. This small region is connected to an area of roughly the same size in the left frontal lobe that, as mentioned previously, regulates the expression of language. Thus, damage to the brain’s left temporal lobe often impairs either a person’s expression or understanding of language, or both. The function of the temporal lobes overlaps in other ways with that of the frontal lobe—including assisting in the control of impulses by more precisely assigning emotional significance to memories. The temporal lobes are also involved in interpreting things we hear, and play a role in visual perception. When the temporal lobes are damaged, one can see why problems might occur.
An injury to the right anterior temporal lobe (that’s the front portion of the right temporal lobe) often results in a phenomena referred to as “prospagnosia” —defined as the inability to recognize familiar faces. Those suffering from this injury can identify that they are looking at a face, and can also identify familiar objects, but they will not be able to identify parents, friends or even a spouse. If, however, a familiar person called them on the telephone, they would be able to recognize the voice and identify the caller.
Joining Memories with Emotion
A very basic summary of the function of the brain’s temporal lobes would include that they are connected to joining memories with emotion and that they are integral to interpreting the meaning of sound and understanding language, as well as forming visual and declarative (factual) memories. Patients with right temporal lobe lesions commonly lose the ability to recognize the significance of musical melodies. Left temporal lobe damage interferes dramatically with the formation, recognition and recall of language. Damage to the temporal lobes, if mild, will likely have either a time-limited effect on these functions (they will quickly heal) or will have no significant effect on function. A moderate injury to the temporal lobes can produce lasting deficits. Genetics, age, state of health and quality and precision of rehabilitation/treatment play the largest role in determining how much function will be recovered. A severe injury to the temporal lobes will likely produce partial or total loss of a number of the functions listed above, and possibly more that have not been covered.
Directly behind the temporal lobes lie the parietal lobes. To the front of the parietal lobes is our somatosensory cortex, which allows us to recognize and recall the form, texture and weight of objects. Another classic medical school case involves lesions on the anterior parietal lobe (the front portion of the lobe), and the resulting phenomenon called “astereognosis” —defined as difficulty recognizing objects by touch. Someone suffering from this disorder will not be able recognize a common object, such as a key, placed in their hand. Portions of the parietal lobes are very important for visual-spatial integration (i.e. awareness of trajectories of moving objects—such as a railroad tie flying at your head) and “proprioception” —defined as the recognition of body parts in space. If you raise your arms above your head, and know that your arms are above your head, this parietal lobe function of yours is grossly intact.
The Dominant Hemisphere
To understand the function of the parietal lobe, and the consequences of injury, it is important to understand what is meant by the term “dominant hemisphere.” The dominant hemisphere is the side of the brain containing the representation of speech and controlling the arm and leg used preferentially in skilled movement. Most often the dominant hemisphere is the left, but in roughly 15 percent of people, the functions of the hemispheres are reversed. The parietal lobe in the dominant hemisphere (usually the left) is responsible for calculation, writing, orientation to left and right, and finger recognition (e.g. “how many fingers am I holding up”). The constellation of symptoms resulting from damage to the parietal lobe in the dominant hemisphere is classically, and famously, referred to as Gerstmann's Syndrome:
The parietal lobe of the non-dominant hemisphere of the brain (usually the right) defines spatial awareness of the opposite (or “contralateral”) side of the body’s position in its environment and is important for skills such as drawing. If there is an injury to the non-dominant parietal lobe, a classic symptom that arises is “hemineglect” —or loss of awareness to the (in most people) left side of the body. More severe damage can produce a phenomenon called “anosognosia” —defined as unawareness of injury or illness. Several years ago I had a patient who was hit on the right side of the head by crane in an industrial accident. He first exhibited spotty awareness of the left side of his body, but quickly deteriorated. One early morning I entered his room to find he had only dressed the right side of his body and only shaved the right side of his face (and I expect he had only brushed the teeth on the right side of his mouth).
How and why did his injury progress from spotty recognition of his left side to total unawareness of it, to the point he didn’t know he had even sustained an injury? Rather than the obvious possibility—bleeding in his brain from trauma, which was effectively ruled-out by completing a ‘STAT’ computed-topography (“CT”) scan of his head— what had first occurred was a severe shearing of a large number of axons in his right parietal lobe. Second, inflammation and cytotoxic changes at the cellular level caused a cascade of damage.
How the Brain Heals
The brain seeks to conserve its resources and optimize its utilization of energy. Its response to an injury is a model for triage in a disaster or combat zone. It first tries to contain the injury, while simultaneously sending a corp of specialized cells to help slow down the deterioration of cells that is rapidly widening in scope. It then “mothballs” cells it finds are not, at present, useful but deemed salvageable, while triggering the feared molecular death signal in those that are not (“apoptosis”). Finally, it starts the process of repairing damaged, but functional, neurons first, and then rebuilding the mothballed—nonfunctional but repairable—neurons. The more severe the injury, or the less able or robust the brain’s emergency cellular response, the more severe and permanent the deficits of function will be.
The occipital lobes, located in the back of your head, are primarily responsible for managing vision—or, more specifically, associating visual stimuli with important memories. Lesions in the primary visual cortex lead to a form of central blindness called Anton's Syndrome: patients become unable to recognize objects by sight, and are typically unaware of that inability. Seizures in the occipital lobe can cause visual hallucinations, often consisting of lines or meshes of color.
The insular lobe integrates sensory information from organs, acting as part of your autonomic nervous system (the automatic processes of the body that we don’t have to think about). It also plays a role in certain language functions, as evidenced in patients that have lesions specific to this area and are completely unable to speak—a disorder termed “aphasia.” The insular lobe also processes aspects of pain and temperature sensation and possibly taste.
The Brain’s “On” Switch
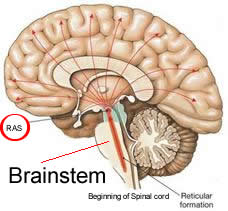
The RAS is located deep inside in the brain. Right below it, a segment of the brainstem called the reticular formation starts to form. It thickens as it extends up toward the RAS, finally branching out into spider web-like circuits that connect to every area of the brain. It takes a great deal of sonic or blunt trauma to really damage the RAS (although it is vulnerable to stroke lesions, cancer masses and birth injuries). Suffice to say, if a brain injury should penetrate to this area, brain function would no longer be an issue. However, in mild-to-moderate brain injuries the web-like projections can be damaged. When the RAS can’t communicate with the brain cells of the upper brain—called the cortex—what results is an under-aroused cortex (note in the diagram above that the arrows project from the RAS to all areas of the cortex—the frontal, temporal, parietal and occipital lobes). This causes difficulty with sustained attention, poor memory, excessive daytime sleepiness and difficulty waking up. In the most extreme cases, it can cause a sustained state of sleep, a vegetative state or even a coma. Conversely, if the RAS is over-excited and arouses either part of, or the entire, cortex excessively, it can cause hyper-vigilance, an excessive startle response, hyper-tactile (touching everything in sight) and hyper-verbal behavior, restlessness and hyperactivity (the RAS and the frontal lobe are often both impaired in ADHD). Simply put, the reticular activating system must activate the respective regions of the brain in a balanced fashion for a person to be awake, alert and appropriately focused.
Types of Brain Injury
When an injury occurs to a specific location in the brain it is called a “focal brain injury.” But injuries do not always impact a single location, or a single area, of the brain. In fact, brain injuries that are more generalized, and non-focal, are the most common. They are termed “diffuse brain injuries,” and often impair functions from multiple areas of the brain. Medicine also has a method of classifying a brain injury as mild, moderate or severe, with further classification into penetrating (e.g. Phineas Gage’s rail tie or a bullet from an enemy soldier), blunt trauma (falling from ladder onto a hard surface) or anoxic injury. Anoxic injury occurs when part of the brain receives insufficient oxygen. A diffuse brain injury usually results from trauma forceful enough that the brain, floating in its pond of spinal fluid, bangs against the inside of the skull. This is referred to as a “coup” injury if it bangs only one side and a “coup-contrecoup” injury if it shifts forcefully in a to-and-fro manner, hitting both sides of the skull. The mechanics of the brain injuries that have been, and are now, occurring in U.S. combat soldiers exposed to blast injuries are often slightly different.
When the brain bumps against the skull, it essentially bruises the brain tissue. But what is most pertinent to brain function occurs at the cellular level, where millions of webs that help form the brain’s communication network are sheared. This phenomenon is referred to, most commonly, as diffuse axonal shear injury (axons are a very important type of nerve cell whose job it is to relay information—an individual axon seen via very high powered microscope is pictured here). If a large number of relay stations are damaged or destroyed, information is either only partly relayed (e.g. fingers only slightly move rather than grasp, words come out as gibberish rather than as the thoughts you wanted to communicate) or not relayed at all (e.g. partial or complete paralysis).
Sound waves propagate force. The more a sound is intensified, the greater its force-wave. I, and several of my colleagues, refer to this as “sonic trauma.” A blast from an improvised explosive device, emitted in close proximity to a soldier/marine/sailor, will produce (if nothing more) enough force to cause a diffuse axonal shear injury. This injury will often manifest as symptoms that can, in part, resemble those of the famous Phineas Gage.
But focal injuries can and do occur, and have been the hallmark of neurological case studies for the past one hundred years.
Learning Brain Research
In 2002 I was involved in a research project with a neurosurgeon that explored the electrophysiology of temporal lobe epilepsy. The neurosurgeon could count on the fact that I would do whatever work he asked, without question, in trade for medical knowledge and the experience of learning the basics of brain research from a renowned medical scientist. One Friday afternoon at 4:00 pm after I had been on-call in the hospital since Thursday at 7:00 am, I was scrubbing in for a case in the neurosurgery operating suite—the most high-tech room in our large university hospital. Robotic arms extended from the ceiling, wall, and floor, allowing a neurosurgeon to sit in a cockpit outside the sterile room and use joysticks to control the arms with a smooth precision that, even a year earlier, was unheard of. Our patient was lying awake in the center of the room, and would remain awake throughout the operation—because the brain tissue itself does not have sensory nerves, local anesthesia is both effective and safer. Just as important, we need him to be awake. All the lights converge on his left temporal lobe. It looks white, with squiggly pink lines traversing its service, disappearing into the crevices (“sulci”) and reemerging over the outfolds (“gyri”) of what was previously described as having the appearance of lasagna.
My attending (the name for the doctor in charge) motioned to me and I asked a nurse, to push the “on” button of a small box that is sitting on a sterile drape over a small roller-cart. A wire sheathed in a sterile tube connected the box to the end of a long, thin needle with a small red button at the base, where a syringe chamber would normally be. We were using that device to test the patient’s visual-language integration capacity by flashing pictures in front of him while giving certain areas of his left temporal lobe an electrical stimulus. His left temporal lobe is the epicenter (or “nidus”) of his nearly intractable seizures. The most effective way to precisely pinpoint areas of the brain that are impaired is through direct stimulation of the tissue. Those areas are precisely marked by recording their position via holographic images created by computer. We were using a relatively new procedure called “multiple subpial resection” —which my attending helped pioneer—to actually dissect axonal communications, effectively stopping the propagation of seizure signals. I heard my patient, Doug, ask, “I saw the zapper box. You guys almost ready to roll?” On a cue from me, a nurse opened a box of large pictures pasted on cardboard.
Doug was thirty-four, well-built and somewhat fearless, as would be expected of a semi-pro bull rider. He had taken a lot of falls prior to an injury he suffered at work, when a guide-arm for a crane struck him in the left side of his head. He began suffering progressively more severe seizures that involved strong abnormal smells, confusing visual recognition of objects, a strong emotion of fear and periodic amnesia. That type of seizure is called temporal lobe epilepsy, and is notably different from the jerking motor seizures most people are familiar with.
We showed Doug an “auto-recognition” picture of a cowboy riding a bull. If he could not visually process this picture and associate the correct name with it, he was likely having a seizure and we would have to immediately stop the operation. My attending signaled that he was stimulating, and I asked Doug what he saw in the picture. There was a pause, “something I miss…a bull and rider…but he has bad form.” His sense of humor appeared to be intact. I picked up a picture of a giraffe and Doug looked perplexed: “Something tall…a stork maybe?” I then picked up a cardboard square with only the word “TRACTOR” in bold, which Doug identified as a “Tractor.” Next was a picture of an elephant, and Doug again responded, “A tractor.” At that, my attending asked me to come around and take a look, and the patient asked me, “You think I’ll ever ride an elephant again?”
Doug’s seizures were markedly better after he received the new operation. But most people who knew him, knew he was different. It wasn’t a completely different Doug, but he was definitely not the same. The fact is, no one is quite the same after suffering a brain injury, be it mild, moderate or severe.
It is evident that traumatic brain injury can completely alter who a person essentially is—your internal self and how you perceive the external world. A less-severe TBI can also leave someone with only mild deficits. What complicates matters so intricately is the vast space between those two poles—where the majority of injuries fall. TBI is certainly far from a new phenomenon. It has, however, become a topic du jour, made prominent by the staggering number of brain injuries suffered by military personnel over the past few years. Be it focal or diffuse, anoxic or sonic, it is essential to know that TBI does not usually announce itself with clear symptoms, such as the crushing chest pain radiating to the shoulder and arm that often signals a heart attack. It most often heralds its presence in bits and pieces, spread both over time and the body, creating a nonlinear picture that can be difficult to fully conceptualize. But with a basic understanding of the different regions and functions of a normal brain, it is possible to open a window and better understand an injured one.
Dr. David Durham is Director of Neuropsychiatry for the Mosaic Neuroscience Group in Santa Fe, New Mexico, and a Clinical Assistant Professor of Psychiatry at the University of New Mexico, School of Medicine.
